5G: How It Works and What It Brings
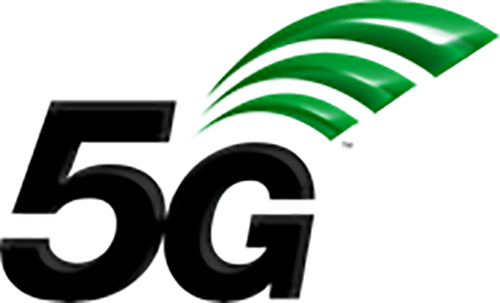
Credit: Wikimedia Commons
Author: Dr. Qiang Lin
Everyone loves a new car, but how many of us think about the number of sensors built into a modern vehicle?
In fact, cars already field more than 100 sensors, according to Dragos Maciuca, executive director of Ford Motor Co.’s Research and Innovation Center in California. That number grows to more than 600 for electric vehicles. All these sensors turn our automobiles into mobile Internet of Things (IoT) devices. With on-board computers providing a vast number of functions, including mobile communication and entertainment systems, most cars today can also perform limited auto-piloting, communicate with nearby cars, and transmit sensor data over the internet. All of these activities are made possible because of increasing deployment of 5G. As introduced by T-Mobile, “the capabilities of 5G, with its high capacity, low latency, network density, and the ability to handle communications among vehicles moving at street and highway speeds, will be essential” for the connected cars of today and tomorrow.
5G is not just a marketing term, but rather the fifth generation of cellular communication technology defined by International Mobile Telecommunications-2020, which defines significant advancements over the fourth-generation standard known as 4G LTE. Similar to 4G LTE, 5G relies on radio signals to communicate with users and IoT devices. Radio signals are transmitted over channels in given frequency bands (each channel has its own frequency allocation within a given frequency band).
As an analogy, think about a teacher instructing a group of students in a classroom. The teacher will try to speak loud enough for each student in the classroom to hear clearly, but this only works for a limited number of students in the classroom. If there are too many students, then the teacher will not be heard. With 5G, to ensure that every device can communicate, a cellular tower, or base station is required to support the number of devices anticipated to be within its service area. In wireless communications, higher frequencies provide higher data rates, but this comes with the price of shorter ranges. In the case of 5G, cellular towers are thus required to be much closer together than previous cellular technologies. Similar to a classroom situation, there are limited numbers of channels in a given frequency band, ranging from a few dozen to several hundreds. Therefore, a limited number of devices can communicate simultaneously with one base station.
- Increase communication distance through radio signal relay, or handoff, from one cell to another
- Increase the total number of communication channels in an area by aggregating all channels from many adjacent cells
- Conserve radio spectrum through reuse.
5G has been designed with different cell sizes to accommodate various user densities – smaller cells for denser population areas, like major urban centers, and larger cells for less populated rural areas, as shown in Figure 1.
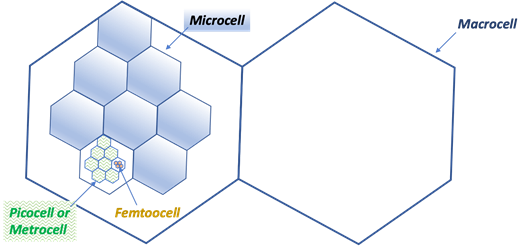
Figure 1 –Micro, Pico, Metro, Femto, and Macro Cells
A microcell covers an area around 4.3 miles wide, supporting 128-256 simultaneous users; and a picocell covers up to 750 feet in a city, supporting up to 64 simultaneous users. A metrocell covers up to 1000 feet, supporting up to 200 simultaneous users. A femtocell covers up to 60 feet, the best option for a home with 4-8 users (imagine having a dedicated cell “tower” in your home), and small offices with 16-32 users. A macrocell covers an area as large as an urban center—approximately 60 miles wide.
When a device is within a given cell, it will communicate through its base station, using either one selected channel (called half-duplex communication; i.e., signal travels in one direction at a time on the selected channel, like a walkie-talkie) or two selected channels (called full-duplex communication; i.e., one channel for transmitting and another channel for receiving simultaneously, like a phone that allows speaking and listening at the same time). As the device moves closer to the cell border, the radio signal gets weaker until it gets to the border of adjacent cell(s). As soon as the device moves into next cell, a handoff of the radio signal occurs, and it will start to communicate with the new base station of the cell it just entered.
5G relies heavily on various frequency bands to deliver unprecedent capabilities for various type of applications and use cases. Pioneering ultra-high speeds and the lowest latencies depend on access to frequencies from 26 GHz to 66 GHz. 5G services also require frequencies below 1 GHz and from 1 GHz to 6 GHz. 5G implementations in the United States are deployed using three frequency bands:
- High-band, 5G only, uses millimeter Wave (mmWave) frequencies with greater bandwidth, able to carry more data in dense urban areas, but requiring cell sites to be in close proximity and having limited penetration in buildings. Verizon’s 5G Ultra Wideband (UWB) network uses 28 and 39 GHz mmWave bands, having 40x bandwidth of 4G LTE 700 MHz network, with download speeds up to 4 Gbps. With mmWave, a 5G base station can employ a new type of antenna, called massive MIMO (Multiple Input, Multiple Output), with more than 100 small antennas on a single radio tower. Massive MIMO will help to deliver peak download speeds of 20 Gbps and connection density of 1 million devices per Km2 goals specified in the International Mobile Telecommunications-2020 (IMT-2020) vision.
- Mid-band, shared by 4G and 5G, uses the 5 GHz spectrum, balancing speed and range, providing broader coverage than high-band, less impacted by buildings, with average download speeds of around 300 Mbps – 7.5x faster than today’s average 4G LTE speeds, with peak download speeds up to 1 Gbps (comparable to cable and fiber-optics speeds from Comcast and Verizon).
- Low-band, shared by 4G and 5G, uses 600 MHz (by T-Mobile), 700 MHz (by Verizon) and 850 MHz (by AT&T) and travels farther than other bands – over hundreds of square miles, passing through more obstacles, and providing a better, more reliable signal both indoors and outdoors.
5G will benefit many areas in our life:
- The three pillars of 5G – ultra low latency, ubiquitous connectivity and massive data capacity – will enable connected, flexible, and responsive manufacturing systems. As manufacturers increasingly create and leverage real-time data on the factory floor, 5G has become a viable choice to deliver secure, isolated network services.
- Among the possibilities to healthcare, 5G transmits large medical images, facilitates telehealth initiatives, and supports remote patient monitoring tools. It also enables more complex uses of artificial intelligence and augmented and virtual reality.
- Faster networks mean smarter homes. 5G allows devices to work free of wires and cables while consuming a minimal amount of power and allowing far more devices to be connected simultaneously than was impossible using older mobile network standards – an essential factor as homes in densely populated areas continue to be filled with a growing number of devices, all hungry for data bandwidth.
- Vehicular communication, vehicle-to-everything (V2X), is one of the critical technologies in intelligent transportation systems to provide connectivity between vehicles, roadside units, and pedestrians. 5G-NR becomes a competitive technology because of extensive coverage, high capacity, high reliability and low delay support.
“AI needs lots of data. IoT generates lots of data. 5G moves that data around. It’s all connected, literally and figuratively,” according to MITRE Vice President Charles Clancy, on the key role 5G plays in making AI and IoT more accessible to Federal agencies and enterprise customers. 5G will be able to cover a wide range of use cases. Consider that 5G will enable better remote healthcare services for veterans, enhanced fleet management for the DoD, full connected sensors in our nation’s battlespaces and for homeland security, and much, much more. MITRE, at the forefront of the 5G innovation, launched an Open Generation Consortium to drive collaboration, breakthroughs, and innovation to envision, design, develop, and demonstrate innovative solutions uniquely enabled by emerging 5G capabilities.
However, as with most new technologies, 5G faces many challenges—cybersecurity is at the top of the list. Although the WIRED story “Hackers Remotely Kill a Jeep on the Highway—With Me in It” was published in 2015, it remains relevant today – nobody wants their cars or smart home IoT devices to be hacked!
Another obstacle is where to set up the plethora of new cell towers required for everyone to access 5G, when each new picocell provides only 1000 feet of coverage, and no one wants one next to their house.
Qiang Lin is an Information Systems Engineer at MITRE and Adjunct Faculty in the Department of Electrical and Computer Engineering at George Mason University. He worked at Deloitte and SAIC previously and has a PhD in Computer Engineering from West Virginia University.
© 2020 The MITRE Corporation. All rights reserved. Approved for public release. Distribution unlimited. Case number 20-3463
MITRE’s mission-driven teams are dedicated to solving problems for a safer world. Through our public-private partnerships and federally funded R&D centers, we work across government and in partnership with industry to tackle challenges to the safety, stability, and well-being of our nation. Learn more about MITRE.
See also:
Will Quantum Computers Revolutionize My Daily Life? Not in the Ways You Might Think
An Update on the Aviation Industry, with Michael Wells and Bob Brents
Interview with Dan Ward, Debra Zides, and Lorna Tedder on streamlining acquisitions
Consequences and Tradeoffs—Dependability in Artificial Intelligence and Autonomy
fleetForecaster Keeps U.S. Aviation System a Step Ahead